The Benefits of Cogeneration
Cogeneration simultaneously produces electricity and beneficial heat from a single fuel source. One example involves capturing waste heat that is a byproduct of coal- and gas-fired power production. The captured heat can be used to heat water or buildings, manufacture products, or create more electricity.
Cogeneration can also involve the capture of waste heat from an industrial or commercial process that is then used to generate electricity, as in the pulp and paper industry.
Cogeneration reduces carbon emissions by displacing the consumption of fossil fuels that would otherwise have been used.
Access Cogeneration Resources from Drawdown Georgia
Market Readiness And Available Technology
Cogeneration technologies and markets are mature and market-ready. Cogeneration systems – also called combined heat and power (CHP) – can be used in individual buildings, in district heating networks, or in manufacturing and electricity generation systems. They have long been viewed as beneficial along a variety of dimensions, including grid reliability, energy efficiency, water conservation, and pollution reduction.
Numerous different types of cogeneration systems are possible, including combinations of (1) prime mover (e.g., microturbine, fuel cell), (2) renewable energy source (e.g., solar PV, wind turbine), and (3) energy storage (e.g., lithium-ion battery, compressed air energy storage). Most experience to date has been with natural gas-driven microturbines (EIA, 2020), but cogeneration with solar systems holds promise.
Several R&D projects focusing on high-efficiency, flexible fuel turbines and district heating integration are being funded by the Department of Energy. These projects aim to improve the efficiency and adaptability of cogeneration technologies, particularly in industrial applications where waste heat recovery can be optimized. A 2016 report by the National Academies categorized the technology readiness level of cogeneration as ranging from prototype to commercial deployment, indicating that while large-scale industrial CHP systems are well-established, smaller-scale "plug-and-play" systems remain underdeveloped.
Cogeneration as a Climate Solution in Georgia
The Drawdown Georgia research team estimates that Georgia could reduce emissions by one megaton (Mt) of CO2e by adding 16 additional 25-MW combined heat and power plants generating electricity with waste heat from industrial processes.
What is the Carbon Emissions Reduction Potential by 2030?
Achievable reduction potential is derived by taking the technical reduction potential, outlined below, and developing a more realistic forecast that takes current rates of deployment, market constraints, and other barriers into consideration.
For cogeneration, the Drawdown Georgia research team has calculated the achievable reduction potential to be 1.4 Mt of CO2e.
What Is the Upper Limit of Carbon Emissions Reduction Potential?
Technical reduction potential reflects the upper limit of emissions reductions for this solution without regard to the constraints that exist in the real world, such as economic or political considerations.
For cogeneration, the Drawdown Georgia research team has calculated the technical reduction potential to be 13 Mt.
Progress on Cogeneration in Georgia
Georgia had 1.4 GW of cogeneration facilities installed in 2017, mainly in the industrial sector, which only increased to 1.5 GW in 2023. At the same time, the number of facilities decreased from 42 to 40. This stagnation is largely due to policy and economic factors. With greater penetration of renewables in Georgia than previously expected, CHP now faces stronger competition from a cleaner grid. Natural gas remains the primary baseload fuel for cogeneration, but its rising costs have impacted economic viability.
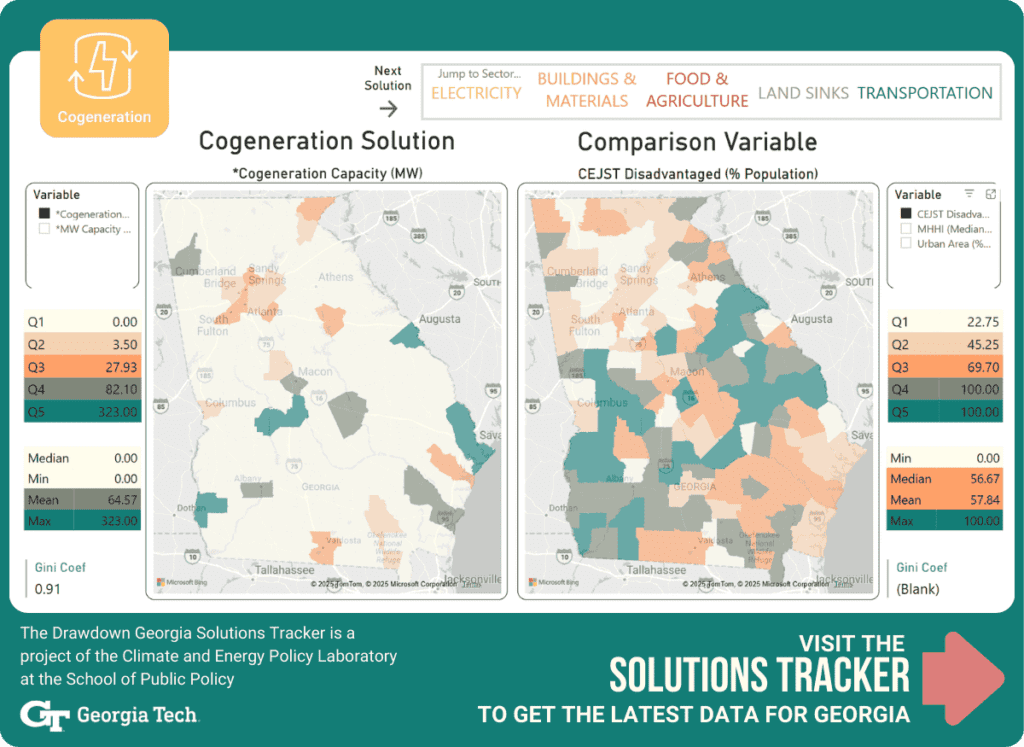
Policy uncertainty also affects CHP deployment. While some states have implemented incentives for cogeneration through clean energy portfolio standards, Georgia has yet to establish a strong policy framework to encourage CHP growth. The lack of consistent financial incentives, combined with relatively low electricity prices, has made it more challenging for industrial facilities to justify new investments in CHP.
Additionally, utilities have been reluctant to integrate excess electricity from CHP facilities into the grid, further limiting growth. These factors suggest that the achievable potential of cogeneration in Georgia may be lower than previously estimated.
Challenges in Scaling Cogeneration
Despite its technical potential, CHP adoption in Georgia faces significant barriers, including economic, regulatory, and market challenges. While CHP can offer substantial energy and emissions savings, the high upfront costs deter many industrial users, particularly smaller facilities that may not have the capital or expertise to develop on-site power generation. Furthermore, utilities have little incentive to support CHP adoption since it reduces their electricity sales, and without regulatory mandates, utilities may continue to resist integrating cogeneration into their resource planning.
Another challenge is that industrial facilities often require long-term certainty to justify CHP investments, but natural gas price volatility and uncertain regulatory frameworks create risk. If natural gas prices continue to rise, CHP may become less competitive against renewable alternatives like solar and wind, particularly as battery storage costs decline. The future of CHP in Georgia will depend on whether policy changes, such as tax credits, streamlined interconnection policies, or utility procurement requirements, can create a more favorable environment for adoption.
How Reliable Is Our Estimate For This Drawdown Georgia Climate Solution?
Using the Georgia Tech - National Energy Modeling System, Brown, Cox, and Baer (2013) estimated that industrial cogeneration had the technical potential to reduce CO2 emissions in the United States by 1.9% by 2035, meeting 18% of U.S. electricity requirements, up from 8.9% in 2012. Given Georgia's sizable and compatible industrial base, a comparable level of penetration would seem achievable. Because of Georgia’s heavy industry, the opportunities for cogeneration should be greater in our state than elsewhere.
Changes to GHG Emissions from Electricity Consumption
Emissions from electricity consumption in the industrial sector, which is the key consumer of cogeneration, have decreased since 2017, but electricity sales have remained almost constant. This suggests that the industrial sector’s carbon footprint for power has reduced.
Cost Competitiveness
Research has documented the cost competitiveness of district, industrial, and power generation CHP systems. The cost-effectiveness of commercial CHP depends on rate design and system ownership (Brown, 2017). Albany CHP LCOE is estimated to be $127-132 (in $2017)/MWh without including a value for the steam that is produced. A 35-year plant life brings the LCOE down to $123/MWh.
The cost competitiveness of CHP systems depends on whether they are customer- or utility-owned and on the type of rate tariff they operate under. Two possibilities have been evaluated:
- a CHP system that is owned and operated by a customer, subject to a flat tariff,
- a CHP system that is owned and operated by a utility subject to time-varying locational marginal prices.
The latter was found to be more financially favorable.
Beyond Carbon Attributes
Beyond reducing greenhouse gas emissions, CHP provides multiple co-benefits. It enhances grid resilience by decentralizing electricity generation, reducing the risk of large-scale outages. Industrial facilities using CHP can operate independently during grid failures, improving reliability for critical infrastructure such as hospitals, data centers, and manufacturing plants. Additionally, CHP can contribute to water conservation, as it often requires less water for cooling than conventional power plants.
However, CHP’s benefits are not evenly distributed. While large industrial players with high heat and power demands can realize significant cost savings, smaller businesses and low-income communities are less likely to access these benefits. Without targeted policies, CHP deployment could widen existing disparities in energy access and affordability.
Moreover, while CHP reduces carbon emissions compared to conventional fossil fuel generation, it still relies primarily on natural gas, meaning it is not a zero-carbon solution. Expanding the role of biomass, hydrogen, or renewable-based CHP systems could help address this limitation.